Hey, everyone! I hope everything's going okay over on your end. Over here, I'm just in pain from all the karate classes and hiking I've been doing (but it's okay, it's a good pain).
We're going to get into a topic that has been one of my biggest interests since we learned about it last year in biology. It's an emerging field in the realm of genetics called "epigenetics," and it's just a fascinating subject to me.
Rewind back to the days of Charles Darwin, who documented and published a work called
On the Origin of Species, which discussed his findings in the Galapagos Islands. He noticed that different islands had species of birds with differently shaped beaks, and formed the earliest theory of evolution.
 |
That one on the top left has seen things. |
Skip forward a bit to Gregor Mendel. He grew a lot of pea plants. Like,
a lot of pea plants.
 |
This many. |
After breeding plants with different traits together, he noticed certain patterns in the generations that proceeded the parent generations.
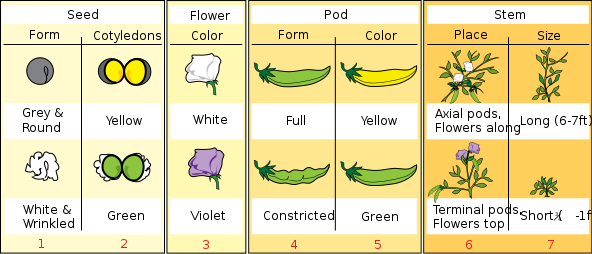 |
These variations. |
This paved the route for a lot of our modern understanding of genetics--that our genes are set, and there's nothing we can do to change them.
OR CAN WE?
 |
*dun dun dunnnnn* |
That's where epigenetics comes in. Basically, epigenetics says that, while you can't change your genes themselves, you
can change how the genes are
expressed.
DNA is wrapped around a molecule called a histone, like this:
 |
Like a little spring! |
This is mostly done to save space, since DNA is so long. A lot of DNA doesn't code for anything, so it stays coiled up in histone groups that are methylated (which just means a methyl group is attached). When it's time for DNA to be transcribed to make stuff, the histones become phosphorylated, which uncoils the DNA.
Epigenetics controls the coiling and uncoiling of the DNA wrapped around these histones.
 |
Awesome! |
Let's bring this back to what I've been studying. There's a section of DNA that codes for the creation of Acetyl-CoA Carboxylase, which creates Malonyl-CoA (which inhibits fat mobilization into the mitochondria). This gene starts transcription from the promoter gene SREBP1, which is actually called "sterol regulatory element-binding transcription factor 1," a name that I hope to never type ever again.
SREBP1 is turned on in the presence of insulin and glucose, which are in high supply in a person with diabetes. Therefore, the cell keeps making Acetyl-CoA Carboxylase, which keeps making Malonyl-CoA, which is kind of causing the problem in the first place!
Fun fact: if you eat sugar, in about a minute, SREBP1 gets turned on, which inhibits the mobilization and use for fuel of fatty acids.
So, not eating a ton of sugar really affects things not only at a cellular level, but at a genetic level as well. And if that's not cool, I don't know what is.